Tissue engineering—optimizing on the human body’s ability to heal itself in order to rebuild muscle and bone—is a major 3D printing application, as it can be used to fabricate the scaffolds that are necessary to support cell regrowth. However, it’s not a magic technology, and there are still some hurdles to overcome, one of which is the fact that making very small, very complex scaffolds is extremely difficult to do. But a team of researchers from Australia’s RMIT University teamed up with clinicians at St Vincent’s Hospital Melbourne to create tiny, intricate bioscaffolds the size of fingernails for tissue engineering purposes using glue and 3D printed molds…not seen quite as often in the medical field.
The team published a study, “Printing between the Lines: Intricate Biomaterial Structures Fabricated via Negative Embodied Sacrificial Template 3D (NEST3D) Printing,” about their research, which took place at the hospital’s bioengineering research, education, and training hub BioFab3D@ACMD. This interdisciplinary collaboration really shows what kinds of innovations are possible when people from different fields put their heads together to solve a problem.
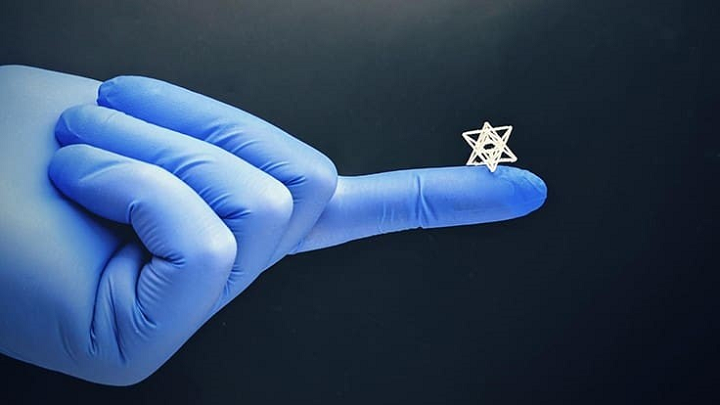
A tiny and intricate biomedical structure created with the new NEST3D technique. (Image courtesy of RMIT University)
“A common problem faced by clinicians is the inability to access technological experimental solutions for the problems they face daily. While a clinician is the best professional to recognize a problem and think about potential solutions, biomedical engineers can turn that idea into reality,” explained study co-author Associate Professor Claudia Di Bella, an orthopedic surgeon at St. Vincent’s Hospital Melbourne.
“Learning how to speak a common language across engineering and medicine is often an initial barrier, but once this is overcome, the possibilities are endless.”
Standard extrusion-based 3D printers would typically not be able to handle this type of print job, so the team flipped the technology on its head. The teeny molds the researchers printed featured complex patterned cavities, which were then filled with biocompatible materials before the molds were dissolved away. According to lead researcher Dr. Cathal O’Connell, a Vice-Chancellor’s Postdoctoral Fellow at RMIT, the team’s biofabrication method used AM technology that’s easily available, which made the process scalable and cost-effective.
Dr. O’Connell explained, “The shapes you can make with a standard 3D printer are constrained by the size of the printing nozzle – the opening needs to be big enough to let material through and ultimately that influences how small you can print.
“But the gaps in between the printed material can be way smaller, and far more intricate.
“By flipping our thinking, we essentially draw the structure we want in the empty space inside our 3D printed mold. This allows us to create the tiny, complex microstructures where cells will flourish.”

NEST3D printed versus FDM printed cubic structures. NEST3D structures made from PCL (45,000 Mn, Sigma-Aldrich, Missouri, USA) and FDM structures printed using white PLA (Ultimaker, Utrecht, Netherlands). FDM structures printed with PVA support (FormFutura, Nijmegen, Netherlands). FDM printing has a limited capacity to make vertical structures at high resolution, the results show the additional deposition of material and stringing effect.
The researchers use PVA glue as the basis for the 3D printed mold in their new method, which they have named Negative Embodied Sacrificial Template 3D, or NEST3D, printing. After the biocompatible material injected into the mold has set, the structure is put in water in order to dissolve the glue, which just leaves the bioscaffold behind.
“Extrusion printing techniques are widely used across tissue engineering and related fields for producing 3D structures from biocompatible thermoplastics, however the achievable structural complexity and porosity can be limited by the nozzle-based, layer-by-layer deposition process. Here, how this limitation can be overcome through a new technique termed Negative Embodied Sacrificial Template 3D printing is illustrated,” the researchers explained in their study. “It is demonstrated how the negative pattern within a 3D printed object can easily describe geometries that are extremely challenging to extrusion print directly with biomaterials, and at high resolution. Negative patterns in a water-soluble sacrificial template can be “developed” by casting in a secondary material and dissolving the template, creating exquisitely complex 3D structures including hyper-branched dendritic structures and open lattices with stiffnesses tuneable over 3 orders of magnitude. The technique is amenable to a plethora of materials from biodegradable thermoplastics (such as polycaprolactone) to resins (including acrylic and epoxy), silicones (including the Sylgard 184 polydimethylsiloxane formulation), ceramics (including hydroxyapatite composites), hydrogels (including agarose and gelatin methacryloyl), low-melt temperature metal alloys and others. Using an unmodified, consumer-grade printer, NEST3D printing achieves high resolution, intricate biomaterial structures with potential applications in biomedical implants and tissue engineering scaffolds.”
As the study’s first author, PhD researcher Stephanie Doyle, explains, NEST3D printing makes it possible to test different combinations of materials in order to select the most effective ones for cell growth.
“The advantage of our advanced injection molding technique is its versatility. We can produce dozens of trial bioscaffolds in a range of materials – from biodegradable polymers to hydrogels, silicones and ceramics – without the need for rigorous optimization or specialist equipment,” Doyle said.
“We’re able to produce 3D structures that can be just 200 microns across, the width of 4 human hairs, and with complexity that rivals that achievable by light-based fabrication techniques.
“It could be a massive accelerator for biofabrication and tissue engineering research.”

Pressure chamber construction and NEST3D printing filling setup. (A-E) construction of the pressure chamber. (A), first an insert is 3D printed using a water dissolvable PVA. (B) standard syringe, 30 cc size. (C) PVA insert placed inside the syringe. (D) PDMS is poured into the syringe (with insert inside) and allowed to cure. (E) pressure chamber with cured PDMS is placed in an ultrasonic bath to accelerate dissolution of the PVA insert. The PDMS is then removed from the syringe, the external surface coated with vacuum grease, and placed back in the syringe. (F-H) sacrificial template filling setup. (F) sacrificial template (the octet truss inside represents empty space) with a curved outer lip to ensure an airtight seal within the pressure chamber. (G) pressure chamber. (H) vacuum grease is applied to outer lip of sacrificial template before being placed as far inside the pressure chamber as possible. The secondary material is added in top and the chamber connected to an air source.
Speaking of materials, Dr. O’Connell noted that while other AM methods are able to create smaller, more intricate biostructures, only specially tailored materials, modified with special chemistry or tuned with specific additives, can be used. The beauty of this process is its materials flexibility.
“Importantly, our technique is versatile enough to use medical grade materials off-the-shelf. It’s extraordinary to create such complex shapes using a basic high school grade 3D printer. That really lowers the bar for entry into the field and brings us a significant step closer to making tissue engineering a medical reality,” Dr. O’Connell said.
Another benefit of NEST3D printing is its ability to create bioscaffolds that work with both cartilage and bone, which could be a major boon for the orthopedics field.
“Our new method is so precise we’re creating specialized bone and cartilage-growing microstructures in a single bioscaffold. It’s the surgical ideal – one integrated scaffold that can support both types of cells, to better replicate the way the body works,” explained Dr. O’Connell.
The researchers say that tests conducted with human cells using their new method have resulted in safe, non-toxic bioscaffolds. Next steps include testing out some new designs to improve cell regeneration, as well as investigating how combinations of biocompatible materials impact cell growth.
Subscribe to Our Email Newsletter
Stay up-to-date on all the latest news from the 3D printing industry and receive information and offers from third party vendors.
Print Services
Upload your 3D Models and get them printed quickly and efficiently.
You May Also Like
Reinventing Reindustrialization: Why NAVWAR Project Manager Spencer Koroly Invented a Made-in-America 3D Printer
It has become virtually impossible to regularly follow additive manufacturing (AM) industry news and not stumble across the term “defense industrial base” (DIB), a concept encompassing all the many diverse...
Inside The Barnes Global Advisors’ Vision for a Stronger AM Ecosystem
As additive manufacturing (AM) continues to revolutionize the industrial landscape, Pittsburgh-based consultancy The Barnes Global Advisors (TBGA) is helping shape what that future looks like. As the largest independent AM...
Ruggedized: How USMC Innovation Officer Matt Pine Navigates 3D Printing in the Military
Disclaimer: Matt Pine’s views are not the views of the Department of Defense nor the U.S. Marine Corps Throughout this decade thus far, the military’s adoption of additive manufacturing (AM)...
U.S. Congress Calls Out 3D Printing in Proposal for Commercial Reserve Manufacturing Network
Last week, the U.S. House of Representatives’ Appropriations Committee moved the FY 2026 defense bill forward to the House floor. Included in the legislation is a $131 million proposal for...