Studying 3D Printed PCL Structures in Tissue Engineering
Researchers from Sweden and Norway are making further strides in tissue engineering, with their recent findings published in ‘Computational and experimental characterization of 3D-printed PCL structures toward the design of soft biological tissue scaffolds.’
As the researchers studied the in-depth effects of mesostructures on the mechanical properties of tissue scaffolds, they 3D printed numerous poly (ε-caprolactone) (PCL)—based scaffolds samples and then analyzed them via finite element analysis (FEA) and computational fluid dynamics (CFD).
Bioprinting continues to offer enormous potential to the medical realm, and research into the fabrication of biocompatible scaffolds—along with new methods to refine them—is a serious topic. Scaffolds must imitate the human extracellular matrix (ECM) comprehensively. This means they must be able to function with excellent properties in the following areas:
- Mechanical
- Transport
- Chemical
- Biological scaffolds
Materials like poly (ε-caprolactone) (PCL) are commonly used in creating medical devices and scaffolds today:
“The semi-crystalline poly (ε-caprolactone) (PCL) is one of the most well-used polyesters owing to its long term mechanical stability but also a low melting point and high thermal stability allowing for a wide processing window in a range of 3D printing techniques,” state the researchers.
“Given PCL’s relatively high stiffness of 300 to 500 MPa, matching the elasticity of native soft biological tissues with 3D-printed scaffolds are challenging in comparison with other conventional techniques, such as salt leaching.”
Mechanical properties can be tuned in a variety of ways, however, via methods like CAD, allowing researchers also to manipulate size, porosity, and more. Image-based design can be used also, allowing for rapid modeling of structures although materials can be limited for meeting high-resolution needs. Other alternative methods such as implicit surface modeling (relying on mathematical equations) and triply periodic minimal surfaces (TPMS) can be effective too despite drawbacks.
The researchers focused on soft biological tissue applications in creating samples, using the following methods for a comprehensive understanding of properties:
- FEA
- CFD
- Experimental material characterization
- SEC
- DSC
- SEM
- Micro-CT imaging
In studying the effects of meso-structures, the researchers noted that while this part of the scaffold also directs nutrients, cell adhesion, and ECM compound deposition, ‘an integral understanding of interactions remains to be developed.’ They note that the meso-structure also influences cell density, due to properties of flow-induced wall shear stress.
“All scaffolds were formed by nine layers and the strand diameter of SD = 0.4 mm,” stated the researchers.
Samples were studied based on the following parameters:
- Strand orientation
- Strand space
- Strand diameter
- Strand length

Schematic illustration of scaffold designs. (a) Building unit for the basic (B) and gradient (G) meso-structures. (b) Building unit for the gradient and staggered (GS) meso-structure. (c) Geometric representation of the computational domain of the virtual bioreactor investigated by computational fluid dynamics (CFD) simulations. (d) Prescription of the displacement d toward loading the scaffold in compression. (e) Prescription of the displacement d toward loading the scaffold in tension.
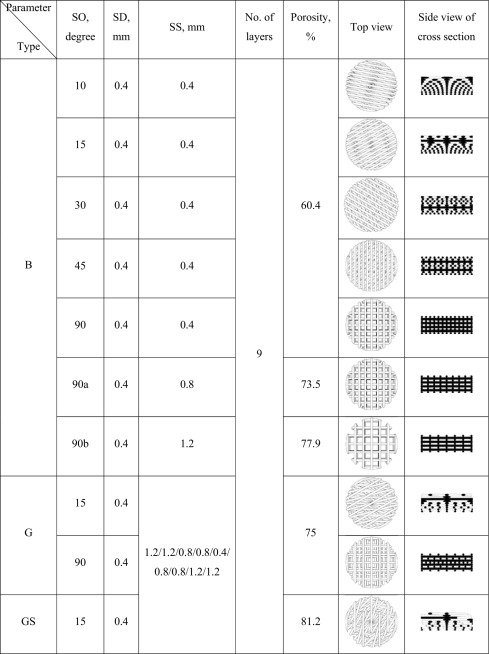
Description of the investigated scaffold designs formed by basic (B), gradient (G) and gradient and staggered (GS) meso-structures. Abbreviations: SD: strand diameter; SL: strand length; SO: strand orientation; SS: strand space.
Designs were grouped as follows:
- Basic
- Gradient
- Gradient meso-structures
- Staggered meso-structures

Mechanical properties of scaffolds using basic (B), gradient (G), gradient and staggered (GS) meso-structures. (a) Finite element analysis-based (FEA-based) elastic moduli of scaffolds under compression and tension. (b) Relation between the compression/tension moduli and the porosity.
“We optimized the printing temperature and speed in order to better replicate the scaffold meso-structure design during printing. However, ambient factors, such as temperature, also need to be carefully controlled toward achieving reproducible scaffold properties. Even minor variations in ambient factors can lead to inhomogeneous solidification of strands and other undesired outcomes,” explained the researchers in the concluding discussion.
“Our results suggest that 3D printing of PCL-based structures is a fully feasible approach to manufacturing soft biological tissue scaffolds. Given their mechanical and transport properties, the GS meso-structure design is the most promising candidate among the designs explored in this study. The biological function of such designed scaffolds should be further studied in bioreactor and/or in-situ studies.”
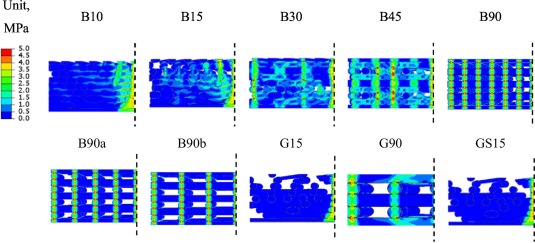
Finite element analysis-based (FEA-based) prediction of the von Mises stress distribution in the strands of scaffolds using basic (B), gradient (G), gradient and staggered (GS) meso-structures. The scaffolds are under vertical compression, and the images represent the stress in the mid-cross section; the dotted line denotes the symmetry line.
As tissue engineering continues to expand, so does the study of and experimentation with scaffolding, from use with electrospinning, bone replacement to improvements in cell viability, and more.
What do you think of this news? Let us know your thoughts! Join the discussion of this and other 3D printing topics at 3DPrintBoard.com.

Top and side views taken from SEM images of 3D-printed PCL scaffolds of basic (B), gradient (G), gradient and staggered (GS) meso-structures.
Subscribe to Our Email Newsletter
Stay up-to-date on all the latest news from the 3D printing industry and receive information and offers from third party vendors.
Print Services
You May Also Like
New Business: Temporary, Migratory, & Modular 3D Printed Architecture
If we look at potentially emerging 3D printing businesses, then architecture has not been fully explored. Yes, there is a lot of house 3D printing going on worldwide. From deployable...
3D Printing News Briefs, April 19, 2025: Material Extrusion Standard, Metal Powder, & More
In today’s 3D Printing News Briefs, we’re covering a proposed standard for material extrusion, before moving on to business and metal powder. We’ll end with a commercial store’s robotic 3D...
Japan Unveils World’s First 3D Printed Train Station
Japan is now home to what we believe is the world’s first train station built with 3D printing technology. Located in Arida City, just south of Osaka, the new Hatsushima...
restor3d Raises $38M to Expand 3D Printed Orthopedic Implants
Backed by $38 million in new funding, restor3d is pushing ahead with the launch of four personalized implant lines, set to roll out in 2025 and 2026. This latest venture...