The bioprinted meat sector is experiencing a surprising boom. While we haven’t seen any widespread deployment of any 3D printed meat or meat substitute, there are a number of pilot products and projects currently underway. There’s even a meat printing startup traded publicly in Israel. And, as this segment begins to flourish and take shape, that means there will be a wider variety of meat types being created—even one of the most luxurious varieties on the market: Wagyu beef.
At Osaka University in Japan, researchers have bioprinted a synthetic version of the expensive beef product, which can cost $200 per pound on average. Part of what makes Waygu so unique is its high intramuscular fat content (commonly referred to as marbling or sashi). Not only is the breed fed for 600 days, which is twice the time as other types of cows, but the animals are also kept in a relaxed environment. In turn, the steak is rich, juicy and has a unique taste and texture.
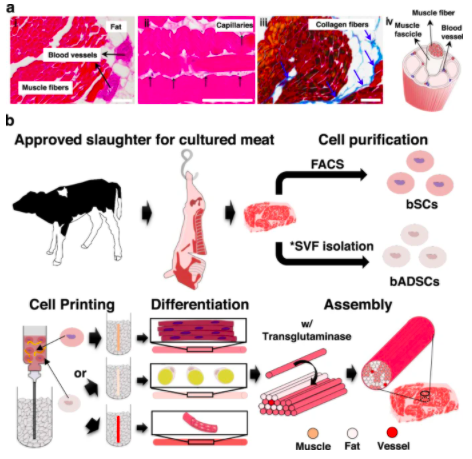
Figure 1 from the Osaka study, overviewing the project. “a Structure of steak. (i, ii) H&E- and (iii) Azan-stained images of a piece of steak. Representative images from three independent experiments are shown. All scale bars denote 100 μm (iv) Schematic of a hierarchical structure in muscle. b Schematic of the construction process for cultured steak. The first step is cell purification of tissue from cattle to obtain bovine satellite cells (bSCs) and bovine adipose-derived stem cells (bADSCs). The second is supporting bath-assisted printing (SBP) of bSCs and bADSCs to fabricate the muscle, fat, and vascular tissue with a fibrous structure. The third is the assembly of cell fibers to mimic the commercial steak’s structure. *SVF stromal vascular fraction.” Image courtesy of Nature Communications.
According to the team, the alt-meat mimics the complex texture of Wagyu beef. To pull it off, the researchers used stem cells from Wagyu cows to 3D print a steak with muscle, fat, and blood vessels that resemble the meat’s marbling. These included two varieties of multipotent cells, bovine satellite cells (bSCs) and bovine adipose-derived stem cells (bADSCs).
“Using the histological structure of Wagyu beef as a blueprint, we have developed a 3D printing method that can produce tailor-made complex structures, like muscle fibres, fat and blood vessels,” said Dr Dong-hee Kang.
The team 3D printed 72 individual fibers of in a “tendon gel” bath of bSCs and bADSCs that would allow for the development of muscle, fat, and vasculature. The individual fibers were then assembled into a structure similar to that of a commercial steak. The tendon gel bath is described more fully below:
“The important feature in the modified SBP, which we have named tendon-gel-integrated bioprinting (TIP), is the introduction of tendon gels to anchor the printed cell fibers for culture. Figure 4a illustrates the process of the TIP in which the printing bath is divided into three parts: the bottom tendon gel, the supporting bath, and the upper tendon gel. G-Gel is used as a supporting bath as described in the above section and the volume of tendon-gels is filled with 4 wt% collagen nanofiber solution (CNFs) which has a reversible sol-gel transition from 4 °C to 37 °C (Supplementary Fig. 12). To separate the layers and maintain the structure we fabricated polydimethylsiloxane (PDMS) wells (Supplementary Fig. 13). After the bSC fiber gelation inside the PDMS well (Supplementary Movie 3), incubation for 2 h at 37 °C induced the supporting bath and tendon gels to become a solution and a gel, respectively, and the PDMS well was then put in the culture medium.”
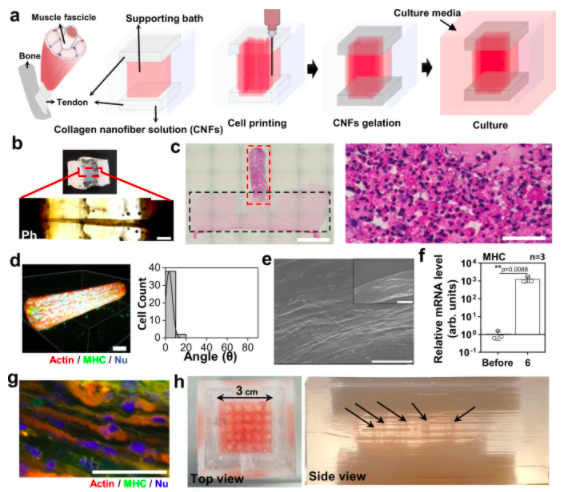
Figure 4 from the Osaka study describing tendon-integrated printing (TIP). “a The schematic of TIP for cell printing. b Optical (upper) and phase-contrast (lower) images of the bSC tissue printed by TIP, keeping the fibrous structure on day 3. The images were taken after fixation. Scale bar, 1 mm. c The H&E-stained image of half of collagen gel (dotted black line)—fibrous bSC tissue (dotted red line) and a magnified image of the fibrous bSC tissue (right). Scale bars, 2 mm (left) and 50 µm (right). d 3D fluorescence image (left) and cell alignment measurement (right) of the TIP-derived bSC tissue stained with actin (red), MHC (green), and nucleus (blue) on day 3 of differentiation. Scale bar, 50 µm. e SEM images of TIP-derived bSC tissue on day 3 of differentiation. Scale bars, 10 µm and 100 µm (inset). f MHC mRNA expression levels of bSCs before printing and TIP-derived bSC tissue on day 3 of differentiation (n = 3 independent samples, pairwise t-test comparison). g Fluorescence image of TIP-derived bSCs tissue stained with actin (red), MHC (green), and nucleus (blue) on day 14 of differentiation. Scale bar, 50 µm. h The optical images of multiple tissue fabrication (25 ea.) by multiple printing. Black arrows indicate printed cell fibers.” Image courtesy of Nature Communications.
The fibers—made up of 42 muscles, 28 fat tissues and two blood vessels—connected together via the tendon gel, resulted in a steak-like meat of 5 mm in diameter and 10 mm in length. Sliced perpendicularly, the meat had a marbling structure resembling Waygu beef.
“By improving this technology, it will be possible to not only reproduce complex meat structures, such as the beautiful sashi of Wagyu beef, but to also make subtle adjustments to the fat and muscle components,” said Michiya Matsusaki, senior author of the Nature Communications study.
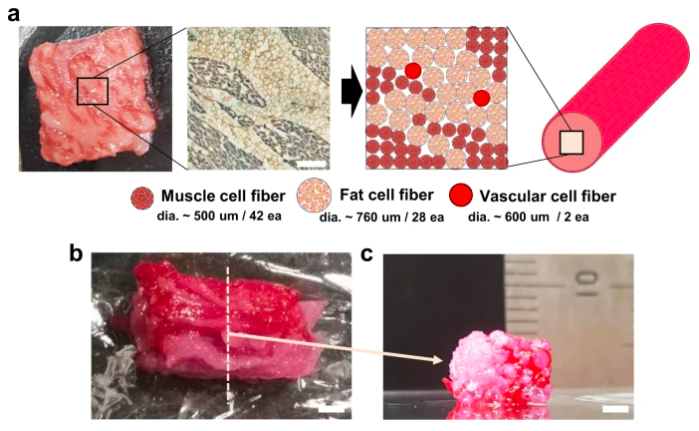
Figure 5 from the Osaka study, overviewing the project. “a Assembly schematic- (right) based sarcomeric α-actinin (blue) and laminin- (brown) stained image (left) of the commercial meat. It is assumed that the diameters of the fibrous muscle, fat, and vascular tissues are about 500, 760, and 600 µm, respectively. Scale bar, 1 mm. b, c Optical images of the cultured steak by assembling muscle (42 ea.), fat (28 ea.), and vascular (2 ea.) tissues at (b) the top and (c) cross-section view of the dotted-line area. Muscle and vascular tissue were stained with carmine (red color), but fat tissue was not. Scale bars, 2 mm.” Image courtesy of Nature Communications.
I’ve never had Waygu beef, but the result doesn’t exactly look appetizing, so I’ll have to take the researchers’ word for it. Regardless, bioprinted meat could potentially play a role in shifting consumers away from naturally derived meat. Though Waygu cows are said to be treated humanely, the large-scale meat industry is generally regarded as a cruel one. Moreover, beef generates 60kg of greenhouse gases per kg of meat produced.
Before relying wholly on the underdeveloped meat bioprinting sector to reduce these emissions, however, it may be worth performing an lifecycle analysis or estimate of the energy footprint we can expect from bioprinted meat at scale. It’s possible that lab-grown meat and industrial 3D printers use significant energy that, at the moment, would be powered by fossil fuels. Alternatives like switching to vegetarianism or veganism or relying on locally produced meat from smaller, organic farms could have a larger impact than inventing an entirely new industrial segment for growing meat in vats.
Subscribe to Our Email Newsletter
Stay up-to-date on all the latest news from the 3D printing industry and receive information and offers from third party vendors.
Print Services
Upload your 3D Models and get them printed quickly and efficiently.
You May Also Like
Reinventing Reindustrialization: Why NAVWAR Project Manager Spencer Koroly Invented a Made-in-America 3D Printer
It has become virtually impossible to regularly follow additive manufacturing (AM) industry news and not stumble across the term “defense industrial base” (DIB), a concept encompassing all the many diverse...
Inside The Barnes Global Advisors’ Vision for a Stronger AM Ecosystem
As additive manufacturing (AM) continues to revolutionize the industrial landscape, Pittsburgh-based consultancy The Barnes Global Advisors (TBGA) is helping shape what that future looks like. As the largest independent AM...
Ruggedized: How USMC Innovation Officer Matt Pine Navigates 3D Printing in the Military
Disclaimer: Matt Pine’s views are not the views of the Department of Defense nor the U.S. Marine Corps Throughout this decade thus far, the military’s adoption of additive manufacturing (AM)...
U.S. Congress Calls Out 3D Printing in Proposal for Commercial Reserve Manufacturing Network
Last week, the U.S. House of Representatives’ Appropriations Committee moved the FY 2026 defense bill forward to the House floor. Included in the legislation is a $131 million proposal for...