Researchers from Australia and China continue the recent trend for exploring the uses of 3D printing and bioprinting for tissue regeneration and wound healing, reviewing the latest research and techniques in ‘Advances in the Research of Bioinks Based on Natural Collagen, Polysaccharide and Their Derivatives for Skin 3D Bioprinting.’
Once damaged, the epidermis can become fragile and must be healed as quickly as possible—especially for older patients. Acting as protection for the whole body, the skin is actually considered an organ, and the largest of them all. While a simple scrape or mild burn may heal quickly, more severe injuries can be fatal, with the authors pointing out in their review that around 300,000 people die each year due to burns—with millions of others suffering through varying levels of treatment. After serious trauma to the skin, many treatments today include:
- Autografts
- Allografts
- Skin substitute
- Cell therapy
- Cytokine therapy
These treatments are often not enough, however, and may result in extended healing time, cost-prohibitive treatment, and other secondary injury. Bioprinting, however, offers positive results in tissue engineering, and especially as vast research continues in countless areas today such as seeding fibroblasts, fabricating scaffolds for assisting in bone regeneration, and investigating a variety of materials for hydrogels. In this review, the authors delved further into specific types of bioink most suitable for skin repair.
“Selecting the appropriate bioink is important as it will influence the overall structure and cellular responses,” explained the authors in the introduction to their paper.
“Specifically, collagen hydrogel is commonly utilized for skin repair, because collagen is the most abundant protein-based natural polymer in skin tissue and is a main component of the native extracellular matrix (ECM), which means it is capable of providing a favorable microenvironment.”
In creating bioinks, biomaterials such as collagen are usually bolstered with blends and composites in an effort to replicate human skin. The challenge is in mimicking:
- Native anatomy
- Physiology of skin
- Surrounding tissue
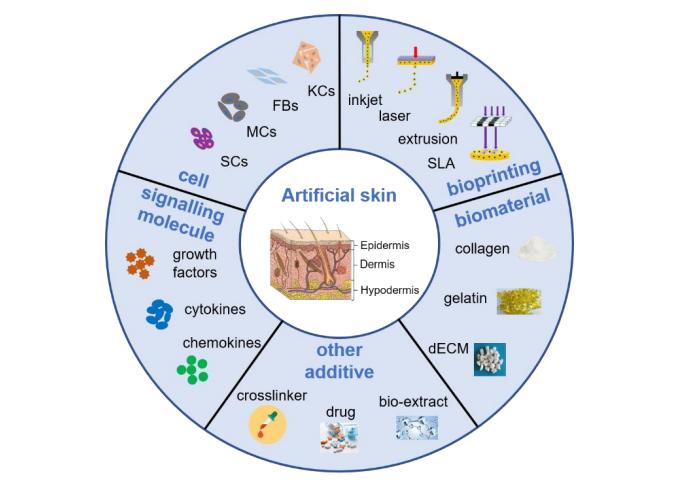
Schematic representation of the main requirements related to 3D bioprinting for skin regeneration. The artificial skin was printed by inkjet, laser, extrusion or stereolithography (SLA) bioprinting technologies with a cell-encapsulating bioink, which consists of biomaterials, constituent cells, stem cells and signaling molecules, or acellular bioink which contains biomaterials only. The mechanical property of artificial skin was enhanced by adding crosslinker, and some drugs or bio-extract were adding to obtain multifunctional skin for wound healing, such as anti-inflammatory and antibacterial. Abbreviations: KCs: Keratinocytes, FBs: Fibroblasts; MCs: Melanocytes, SCs: Stem cells, dECM: Decellularized extracellular matrix
3D printed skin can be applied to the wound itself or cultured for transplantation later.

The basic process of 3D bioprinting skin. Various cells like keratinocytes, fibroblasts and melanocytes are collected from the patient and cultured in a cell culture system. A suitable biomaterial is mixed with the cells and the formed bioink is fed to the bioprinting system. Then the skin is printed with appropriate 3D bioprinting technology according to the 3D pattern that is captured from the wound using CAD/CAM approaches. The printed skin could be directly printed to the wound surface or cultured under appropriate conditions to obtain mature skin for transplantation. Adapted from [22] under open access license.
- Inkjet bioprinting
- Laser bioprinting
- Extrusion bioprinting
- Stereolithography bioprinting
- Microfluidic bioprinting
In most bioprinting, researchers seek printability, biocompatibility and lack of toxicity, good cell adhesion, and suitable mechanical properties. Collagen is often used due to its similarity to skin; however, mechanical strength and cell adhesion are often inferior, leaving researchers to employ additives.
Other materials are often used too, such as:
- Gelatin
- Alginate
- Fibrin
- Hyaluronic acid
- Chitosan
- Pectin
As the bioink must imitate the ECM as much as possible, researchers must often adjust it for better sustainability of cells.

Decellularized extracellular matrix (dECM) bioink for skin bioprinting. (A) Qualitative analysis with H&E, Masson’s trichrome and DAPI staining (left) and quantitative analysis (right) of skin-derived dECM bioink, including collagen, GAGs, elastin, hyaluronic acid and DNA, which indicated that it successfully removes the cellular components from the native skin tissue. (B) Representative photographs (left) of 3D cell-printed in vitro skin equivalents, electrical resistance values for each group (S-HSE is the group using skin-derived dECM bioink, and the others are control group) (middle) and protein expressions (right) of the S-HSE group on day 10 after ALI culture. Involucrin (IVL): Early epidermal differentiation marker; keratin 10 (K10): Late epidermal differentiation marker. (C) Representative photographs of skin wound tissues on day 21 (wound gap, black lines exhibit distances between advancing edges of wounds) (left) and wound areas relative to the orginal ones, indicating the bioprinted skin based on dECM bioink accelerated wound healing. Reproduced with permission from [122]; copyright Elsevier, 2018. (D) Photographs of the upper and bottom heating modules installed in 3D printing equipment (left), and conceptual diagram of non-heating and heating conditions (right). (E) Qualitative (bottom) and quantitative (upper) analysis of cellular activities under different heating conditions. activities under different heating conditions. Reproduced from [23] under open access license.
“Despite advancements with the use of skin cells (keratinocytes, fibroblasts and melanocytes) and multipotent stem cells (ADSCs, MSCs) in bioprinting of skin tissue constructs, the use of induced pluripotent stem cells (iPSCs) may provide more opportunities in skin bioprinting” concluded the researchers. “Moreover, advancements in skin bioprinting may also come with the co-culturing of various cells that are usually found in the skin layers and related tissues including keratinocytes, fibroblasts, pericytes, neural cells and ligament cells.
“In the future, it is critical to engineer fully functional skin by bioprinting structures that closely mimic the native anatomy and physiology of skin and surrounding tissue.”
What do you think of this news? Let us know your thoughts! Join the discussion of this and other 3D printing topics at 3DPrintBoard.com.
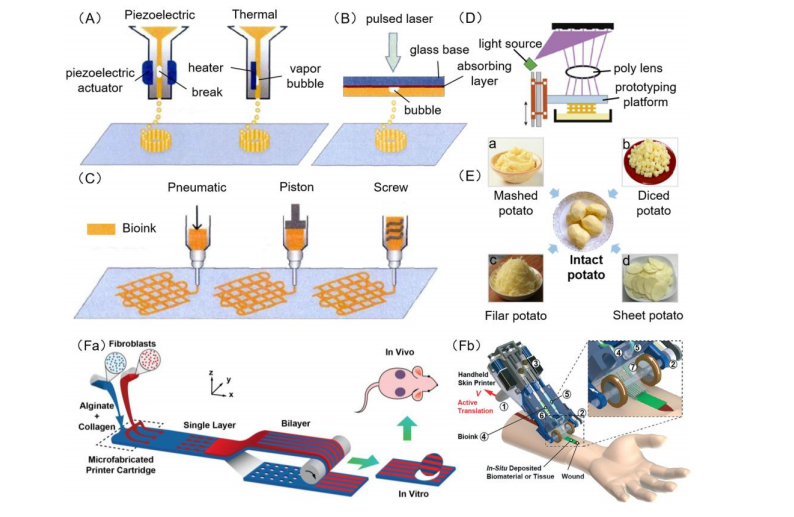
Schematic diagram of 3D bioprinting. (A) Piezoelectric inkjet bioprinters form pulses by piezoelectric pressure to force droplets from the nozzle, while thermal bioprinters use air-pressure pulses produced by a printhead that is electrically heated. (B) Laser bioprinters use laser focused on an absorbing substrate to generate pressures that propel bioink onto a collector substrate. (C) Extrusion bioprinters use pneumatic, piston or screw dispensing systems to extrude continuous beads of bioink. (D) Stereolithography bioprinters use a digital light projector to selectively crosslink bioink plane-by-plane. Images (A)–(D) were adapted with permission from [66]; copyright Journal of Zhejiang University (Engineering Science), 2019. (E) Schematic diagram of traditional 3D bioprinting the reverse process of cutting potatoes; laser (B), inkjet (A), extrusion (C), stereolithography (D) bioprinting seem like the assembling of potatoes from mashed potato (a), diced potato (b), filar potato (c) and sheet potato (d), respectively. (F) Microfluidic bioprinters use a microfluidic-based device to extrude a biopolymer sheet with precise spatio-temporal control over the component proportion of bioink. (a) Illustration of proposed skin printer for formation of cell-populated skin substitute in a microfabricated printer cartridge and application in vivo. Image (Fa) was reproduced with permission from [51]; copyright Chemical and Biological Microsystems Society, 2013. (b) Rendere image of handheld bioprinter. O1 a handle, O2 a stepper motor to define the deposition speed, O3 two on-board syringe pump modules controlling the flow rates of bioink and cross-linker solution, O4 bioink, O5 cross-linker solution, O6 syringe holder, O7 3D printed microfluidic cartridge for spatial organization of solutions and sheet formation. Image (Fb) was reproduced with permission from [65]; copyright Royal Society of Chemistry, 2018.
Subscribe to Our Email Newsletter
Stay up-to-date on all the latest news from the 3D printing industry and receive information and offers from third party vendors.
Print Services
Upload your 3D Models and get them printed quickly and efficiently.
You May Also Like
Making Space: Stratasys Global Director of Aerospace & Defense Conrad Smith Discusses the Space Supply Chain Council
Of all the many verticals that have been significant additive manufacturing (AM) adopters, few have been more deeply influenced by the incorporation of AM into their workflows than the space...
EOS in India: AM’s Rising Star
EOS is doubling down on India. With a growing base of aerospace startups, new government policies, and a massive engineering workforce, India is quickly becoming one of the most important...
PostProcess CEO on Why the “Dirty Little Secret” of 3D Printing Can’t Be Ignored Anymore
If you’ve ever peeked behind the scenes of a 3D printing lab, you might have caught a glimpse of the post-processing room; maybe it’s messy, maybe hidden behind a mysterious...
Stratasys & Automation Intelligence Open North American Tooling Center in Flint
Stratasys has opened the North American Stratasys Tooling Center (NASTC) in Flint, Michigan, together with automation integrator and software firm Automation Intelligence. Stratasys wants the new center to help reduce...