Ultimaker 3D Printer + Laser Microfabrication + Stem Cells = Biodegradable Hard Tissue Implants
Currently FFF-based 3D printers are used for fabricating objects, and femtosecond (ultrashort pulsed) laser induced ablation (selective material removal by instant evaporation) is employed for precise material microfabrication. What if we combine the best of both of these two worlds? Why not to use 3D printed structures as three-dimensional substrates for laser manufacturing? Widely used PLA material is biodegradable, thus manufacturing of microporous structures enables the creation of 3D custom made bioresorbable implants.
Vilnius University Laser technology and biochemistry scientists joined forces to combine straightforward fused filament fabrication (FFF) 3D printing with advanced direct laser writing (DLW) for the manufacturing of biodegradable scaffolds with sub-cellular spatial precision. Such microporous scaffolds fabricated from PLA were seeded with rabbit stem cells and initial tests revealed not only sufficient biocompatibility, but also efficient proliferation and a trend to differentiate appropriately. This shows great potential in biomedical surgery applications, such as restoration of hard tissue (reconstruction of bone) or soft tissue (hernia treatment). So, how can this be practically realized?
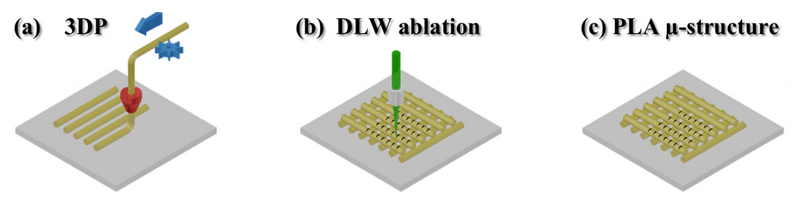
Figure 1. The schematic principle of fabrication by combining FFF and DLW techniques: (a) – 3D structure is printed by melting and extruding a material through a nozzle; (b) – secondary structuring is realized employing DLW; (c) – the final PLA microporous biodegradable scaffold.
First of all – a simple commercial “Ultimaker original” 3D printer applying PLA thermoplastics is used for the manufacturing of objects with internal three-dimensional geometry (Fig. 1 and 2). Then an amplified femtosecond laser is employed for the precise microstructuring of printed template’s (Fig. 3). If needed, the samples are immersed into an acetone bath to generate roughness at the nanoscale (Fig. 4-7) and increase the sample’s hydrophilic properties desired for the improved cell adhesion.
Prior to biocompatibility studies, the scaffolds are inspected with a scanning electron microscope (SEM). And finally, stem cells are seeded, after their viability and behavior are investigated under a fluorescence microscope (Fig. 8). The results were very promising, such scaffolds can be clinically inserted as implants and depending on their biodegradable properties disintegration would take place in the body within period of 2 years or so, thus giving enough of time for the cells to overgrow the constructs and form the required hard tissue. Currently, such surgical treatment is performed using titanium implants which are biocompatible but stay in the body for the lifetime. Another issue is that traditional implants are designed just for the replacement of bone inside the body, whereas the proposed novel biodegradable scaffolds could provide a tissue regenerative medium (known as ECM – extracellular matrix) on the boundary of hard-soft tissue.
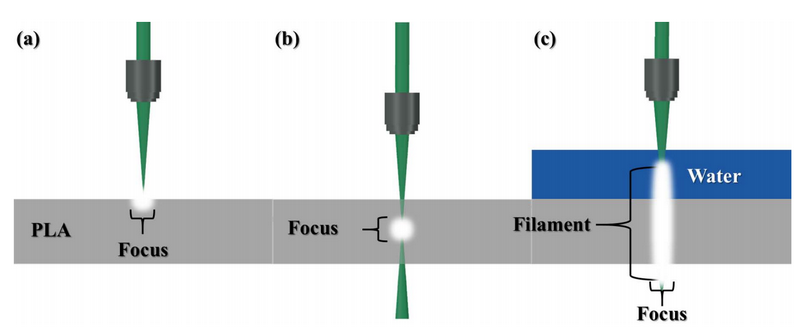
Figure 2. Various laser beam focusing approaches: (a) – standard focused beam to the surfaceof transparent/opaque substrate; (b) – focused beam in the bulk of the transparent material, this is possible using ultrashort pulses; (c) – FPF in liquid assisted rapid microfabrication of transparent/opaque targets, this is exceptional novel approach where water serves as a media for filament formation and ablated debris removal.
Special attention should be given so that the laser processing includes a special femtosecond pulse filament (FPF) assisted technique. It is based on a high repetition ultrafast laser (in the studied case – 200 kHz and 300 fs = 200 000 pulses per second where single pulse duration is 300*10^-15). Once such beam is focused into a water and creates light filament which has a homogenous intensity distribution along the propagation direction. Note, that the light intensity (amount of photons carrying energy per second to the area) equals 75 TW/cm^2 which is greater than Kashiwazaki-Kariwa, the largest nuclear power station, which produces 7.965 GW of electrical output.
Of course, one needs to have in mind that the pulse is ultrashort and tightly focused, thus the average optical power of the employed laser is just 5 Watts. Anyhow, these high powers and short durations are not just extreme numbers, but are a necessity to induce an effect known as light filamentation and provide precise optical structuring of materials without any undesired thermal damage. It means the light matter interaction occurs much more rapidly before the material starts to melt and evaporate. This feature is unique and empowers unique precision and negligible material damage around the affected zone.
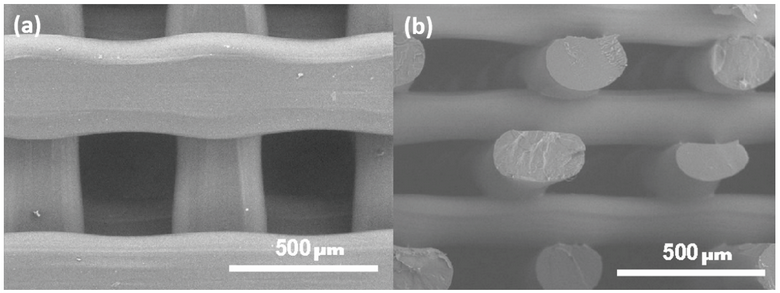
Figure 3. SEM micrographs of printed PLA – micro-logpile (woodpile) structure: (a) – top view, latteral period 700 μm and line widths of 300 μm separated by 400 μm gaps, (b) – side view, axial period was 400 μm and line heights of 200 μm separated by 200 μm gaps.
Additionally, a 3D printer can be employed for the creation of wavy surfaces by simply alternating a sample’s height (Fig. 4 (a,b)). It results in the fabrication of periodic wavy grating which are attractive surfaces for cell migration and guiding. Some researchers could comment that this is a well known fact!? Yes indeed, but the novelty lies in the fact that such custom made biocompatible wavy surfaces at a miscroscale is straightforwardly made with a desktop 3D printer pricd at around $1000, a cost which is usually paid for by a single custom made implant.

Figure 4. SEM micrographs of fabricated microgrooves in PLA employing DLW and sharpbeam focusing conditions: (a), (b) – wavy sheet, (c), (d) – log-/wood-pile structure at different magnifications, front and side views, respectively.

Figure 5. SEM micrographs of microholes drilled in PLA sheet (left) and woodpile structure (right): (a,c) – using sharp focussing; (b,d) – using femtosecond pulse induced light filament.

Figure 6. SEM micrographs of femtosecond pulse filament assisted cut shapes: (a) and (c) – bulk, (b) and (d) – woodpile-scaffold type.
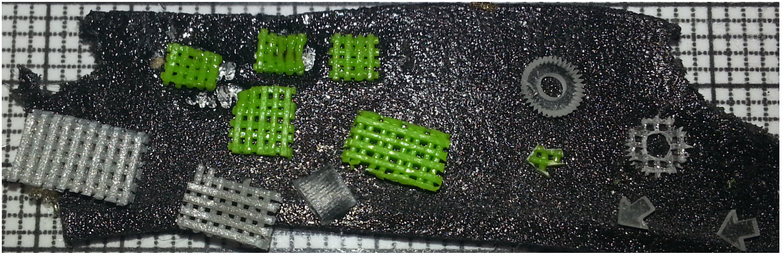
Figure 7. Various colors and shapes microstructure samples processed and obtained via DLW sharp beam focusing and FPF assisted fabrication shown as seen by a naked eye. The reference grid squares corresponds to 1 mm.
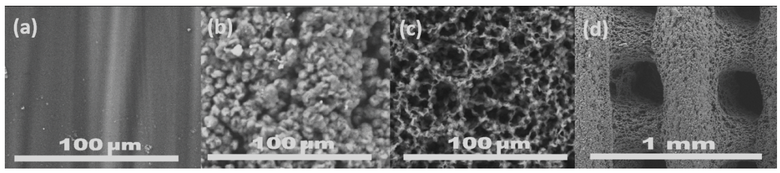
Figure 8. SEM images of a plain PLA surface (a), and after 1 and 8 minutes of immersion in acetone, (b) and (c), respectively. A woodpile structure after 1 minute in acetone (d).
However, the presented method is not limited to applications in biomedicine. It can serve as a tool for creating components in microfluidics and micromechanics. Precise control of porosity (filling ratio), surface area and surface roughness enables the creation of mesoscale structures (mesoscalestructures have dimensions of several millimeters or centimeters with distinguishable and functional features down to micrometer and nanometer scale; thus, the structures cannot be simply defined as microstructures). Such objects have physical, chemical and biological properties which are not limited by the bulk properties of the material.
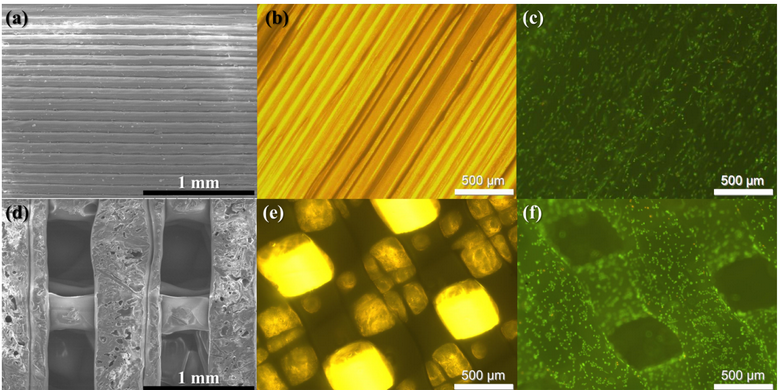
Figure 9. The SEM (a, d), light (b, e) and fluorescence microscope images (c, f) of the 3D printed scaffolds and myogenic cells grown on them: top – 2.5D full-filled, bottom – 3D microporous; in (c), (f) cells are grown for 24 h and stained by an acridine orange-ethidium bromide mixture. Alive cells are stained green and dead cells should be stained orange (there are no dead cells). The surface of the material is evidently biocompatible. The myogenic cells on the scaffolds were situated and elongated along the fibers. The phenomenon of special cell arrangement could be attributed to cell differentiation potency.
Future work is targeted towards chemical modification of pure PLA material for enhanced biological properties, mechanical tests of such 3D microporous scaffolds and their preclinical applications with laboratory animals. Authors of the work are open for possible cooperation to implement this technique into Lab-on-Chip (LOC) and Micro-Total-Analysis-Systems (μ-TAS). More details can be found in a freshly published peer reviewed scientific article at an open access peer reviewed journal MicroMachines: https://www.mdpi.com/2072-666X/5/4/839. Discuss this report in the 3D printed hard tissue implant forum thread on 3DPB.com.
M. Malinauskas, S. Rekštytė, L. Lukoševičius, S. Butkus, E. Balčiūnas, M. Pečiukaitytė, D. Baltriukienė, V. Bukelskienė, A. Butkevičius, P. Kucevičius, V. Rutkūnas, S. Juodkazis, 3D Microporous Scaffolds Manufactured via Combination of Fused Filament Fabrication and Direct Laser Writing Ablation“, Micromachines 5, 839-858 (2014).
The report was prepared by Vilnius University scientists Dr. Mangirdas Malinauskas (Laser Research Center) and Dr. Virginija Bukelskienė (Institute of Biochemistry).
Subscribe to Our Email Newsletter
Stay up-to-date on all the latest news from the 3D printing industry and receive information and offers from third party vendors.
Print Services
You May Also Like
New Business: Temporary, Migratory, & Modular 3D Printed Architecture
If we look at potentially emerging 3D printing businesses, then architecture has not been fully explored. Yes, there is a lot of house 3D printing going on worldwide. From deployable...
3D Printing News Briefs, April 19, 2025: Material Extrusion Standard, Metal Powder, & More
In today’s 3D Printing News Briefs, we’re covering a proposed standard for material extrusion, before moving on to business and metal powder. We’ll end with a commercial store’s robotic 3D...
Japan Unveils World’s First 3D Printed Train Station
Japan is now home to what we believe is the world’s first train station built with 3D printing technology. Located in Arida City, just south of Osaka, the new Hatsushima...
restor3d Raises $38M to Expand 3D Printed Orthopedic Implants
Backed by $38 million in new funding, restor3d is pushing ahead with the launch of four personalized implant lines, set to roll out in 2025 and 2026. This latest venture...