TU Delft Researcher Wins ISSMO/Springer Prize for Space-Time Topology Optimization in Additive Manufacturing
Researchers from TU Delft have not only developed a new method for optimizing structures and their fabrication sequences, but the main author has also won the ISSMO/Springer prize from the International Society of Structural and Multidisciplinary Optimization (ISSMO), due to the impressive ‘Space-Time Topology Optimization for Additive Manufacturing: Concurrent Optimization of Structural Layout and Fabrication Sequence,’ recently published in the Structural and Multidisciplinary Optimization journal.
Dr. Weiming Wang has been awarded the prize for his research, which he delivered a presentation on at the 13th World Congress of Structural and Multidisciplinary Optimization (WCSMO) in May 2019 in Beijing. Wang will be formally awarded the ISSMO/Springer prize at the General Assembly of WCSMO-14 in 2021. This work, co-authored by Dirk Munro, Fred van Keulen, Charlie Wang, and Jun Wu, received attention due to its innovative strength, simultaneously allowing for structural optimization and fabrication sequences.
Robotic-assisted additive manufacturing processes play a major role in this new work, involving two design variables: a density field that defines the layout, and a time field for generating intermediate structures. The structures that require optimization may be extremely complex, requiring ‘additional constraints’ to allow for manufacturing and construction; however, those very constraints can actually jeopardize structure.
The researchers point out that great progress has been made in these areas but until now optimization and structural fabrication have not been put together in a simultaneous exercise.
“The proposed method forms a perfect match with recent advances in additive manufacturing which enable flexible fabrication beyond consecutive planar layers,” explain the authors.
Space-time topology optimization includes assessment of the overall, completed structure—but includes the ‘incomplete’ portion of the process, as well as intermediate phases.
To imagine this new system being used, the researchers suggest the idea of a bridge being built with robotics. As the ‘heavy robots’ move along, they are able to ensure not only that the bridge is strong, but that it can withstand the weight of the robots during fabrication too as they are deliberately supported.
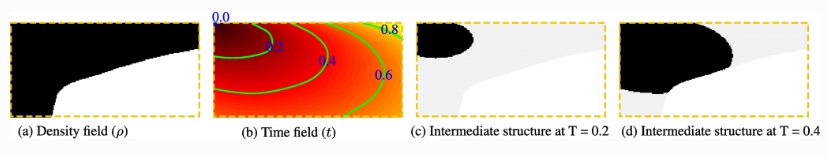
Illustration of a discrete density field (a), a continuous time field (b), and resulting intermediate structures at T = 0.2 (c) and T = 0.4 (d). In (c) and (d), for illustration purposes, in gray the full structure is shown. The outer contour of the design domain is indicated by the orange dashed lines.

Illustration of the density field (bottom), the time field (top), and the corresponding filtering and projection operations for specifying an intermediate structure (right)
To prevent isolated material patches from forming, the researchers focused on improving the incremental AM process, where one layer is to be deposited onto the next.
“An isolated material patch during the fabrication process can be associated with a local minimum in the time field; all its adjacent elements have a larger time value and thus will be fabricated later,” explained the researchers.

Time fields and structures generated with different manufacturing startpoints (blue regions). From top to bottom: the initial time fields (constructed from distance fields corresponding to the startpoints), optimized time fields, and optimized structures. The black curves indicate the boundaries between adjacent parts which have been manufactured during different manufacturing stages. The number of stages, 8 in this test, is not a design variable but is prescribed. Consequently, Ti, the values which are used to segment the time field are determined. The colorbar on the right indicates the time values. (This colorbar is used consistently in this paper for other figures as well)
The self-weight of the platform has an effect on the structure as it grows, and during the AM process, there may be substantial deformation with materials such as thermoplastic polyurethane.

A bridge to be fabricated by a robotic platform. The bridge under construction shall support the movable robotic platform at all intermediate stages. Image courtesy of MX3D (www.mx3d.com)
Four different weighting factors (αi = 0.001, 0.1, 0.4, and 0.6) were used in optimization, with the researchers noting that ‘as the influence of self-weight increases, the solutions are characterized by an increased number of solid elements in the vicinity of the fixing location (left edge).’ The design domain was separated into 12 parts, leaving the research team to figure out the number of solid elements for each subdomain.
The robot should be working in consecutive motion, beginning with the top left corner and then stepping to the right, placing material within its range, for each area. If the process is moving along smoothly, the same amount of material should be deposited in each area as the speed should be same—with process dependent loads in place using process time-dependent materials.
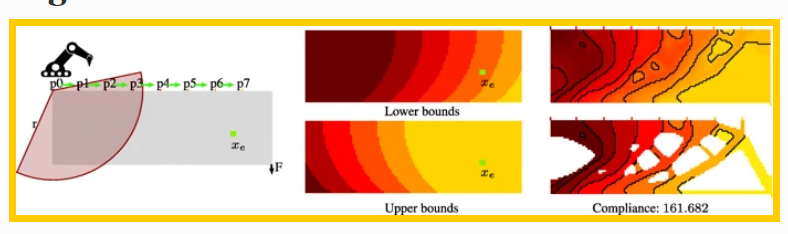
Left: A robot printer platform moves along the structure from left to right on the top (left). Middle: The lower and upper bounds of the manufacturing time. Right: The optimized time field, and the optimized density field colored with the time field. The number of manufacturing stages is 8 and αi = 0.5. The compliance of the final structure is listed at the bottom
“Clearly, these examples are by no means exhaustive with respect to the potential of the formulation. The convergence and influence of some key parameters are evaluated by an extensive parameter study,” conclude the researchers.
“The proposed formulation opens up a new direction in the integration of topology optimization and advanced manufacturing techniques. Extending this formulation from 2D to 3D is straightforward. As future work, we are particularly interested in considering manufacturing introduced distortion which highly depends on the manufacturing sequence.”
While this novel concept stands out as highly innovative, researchers today are incorporating robotics into much of their work, from taking manufacturing to the next level in 4D to integrating soft mechanical materials, sensors, and more. What do you think of this news? Let us know your thoughts! Join the discussion of this and other 3D printing topics at 3DPrintBoard.com.
[Source / Images: TU Delft]Subscribe to Our Email Newsletter
Stay up-to-date on all the latest news from the 3D printing industry and receive information and offers from third party vendors.
You May Also Like
Gorilla Sports GE’s First 3D Printed Titanium Cast
How do you help a gorilla with a broken arm? Sounds like the start of a bad joke a zookeeper might tell, but it’s an actual dilemma recently faced by...
Nylon 3D Printed Parts Made More Functional with Coatings & Colors
Parts 3D printed from polyamide (PA, Nylon) 12 using powder bed fusion (PBF) are a mainstay in the additive manufacturing (AM) industry. While post-finishing processes have improved the porosity of...
$25M to Back Sintavia’s Largest Expansion of Metal 3D Printing Capacity Since 2019
Sintavia, the digital manufacturing company specializing in mission-critical parts for strategic sectors, announced a $25 million investment to increase its production capacity, the largest expansion to its operations since 2019....
Velo3D Initiates Public Offering in a Bid to Strengthen Financial Foundations and Drive Future Growth
Velo3D (NYSE: VLD) has been among a number of publicly traded 3D printing firms that have attempted to weather the current macroeconomic climate. After posting a challenging financial report for 2023,...