In the recently published ‘Engineered 3D Polymer and Hydrogel Microenvironments for Cell Culture Applications,’ authors Daniel Fan, Urs Staufer, and Angelo Accardo explore the world of bioengineering and microenvironments, reviewing the best types of methods, materials, and challenges that must be met.
Material properties are one of the most highly discussed topics today in 3D printing, and this is the area the authors choose to delve into first. Stiffness and rigidity may be critical in materials for some projects, and as researchers attempt to mimic properties of the extracellular matrix.
“The fine control of mechanical properties of the employed biomaterial provides therefore a crucial tool for guiding the fate of stem cells whose final differentiation can be widely tuned as demonstrated, for instance, in human mesenchymal stem cells (hMSCs), able to differentiate into neural, muscle, or bone lineages after being cultured on polyacrylamide gels of varying stiffness (≈0.1–1, 8–17, or 25–40 kPa respectively), and neural stem cells differentiating into neurons or glial cells in the presence of softer (≈1 kPa) or stiffer (≈10 kPa) collagen/hyaluronic acid matrices respectively,” stated the researchers.
Roughness and porosity must be manipulated too for influencing cell adhesion and proliferation, allowing them to wrap around structures as well as becoming patterned in a variety of topologies. The void regions afforded by suitable levels of porosity mean that tissue can receive nutrients and oxygen.
“Often, pristine materials are not equipped with the level of porosity required in tissue engineering or drug-screening applications. This may lead to the creation of a non-efficient artificial ECM that prevents the desired cell proliferation, differentiation, and migration mechanisms. To address this problem and guide a fine tuning of the porosity, several fabrication and characterization techniques can be employed involving, among other things, salt leaching, freeze-drying, stereolithography (SLA), scanning electron microscopy, and micro-computed tomography imaging,” explain the authors.
And while biocompatibility is one of the most obvious necessities, it can also be one of the most challenging areas of tissue engineering. In some cases, scaffolding must be biodegradable also—this is necessary in targeted drug delivery as the vehicle must ‘fade away’ without leaving any toxins in the body.
3D printing itself—and especially bioprinting—can be achieved in myriad ways today as manufacturers, designers, engineers, researchers, and users around the world have created software and hardware to fit their project requirements, to include:
- Selective laser sintering and binder jetting
- FDM 3D printing
- Extrusion-based bioprinting
- Light-assisted additive manufacturing processes
With these techniques, hybrid methods have also become increasingly popular, combining different types of fabrication processes according to need.
“Extrusion of rigid PCL was combined with SLA printing of soft poly(ethylene glycol) diacrylate (PEGDA) to quickly build composite scaffolds with pore sizes of about 350 µm in diameter, improving the hydrogel rigidity as well as viability of cells. Yet another example of a hybrid method to form hierarchical structures is to use FDM combined with gas foaming: a poly(vinyl alcohol) (PVA)/PLA blend was deposited via FDM, followed by gas foaming to form micropores of ~10 µm diameter,” explained the researchers.
While most digital fabrication calls for specific and sometimes new or unique materials, bioprinting is driven by the need to keep cells alive. The entire process can be uncertain and fragile, and materials must fit the research project perfectly to meet success.
“By combining, mixing, and hybridizing different materials with vastly different properties, specific functions such as biocompatibility, biodegradability, mechanical stiffness, structural strength, cytotoxicity, and bioactivity can be tuned,” explain the researchers.
Typical materials include:
- Thermoplastics
- Polycaprolactone (PCL)
- Polypropylene Fumarate (PPF)
- Polylactic Acid (PLA)
- Polyether-Ether-Ketone (PEEK)
- Hydrogels
- Polyethylene Glycol (PEG)
- Polysaccharides
- Chitosan
- Alginate
- Silk
Technique and materials must be perfectly coupled for success in the delicate process of bioprinting but is often connected to the availability of the proper materials. There are other options available now too such as scaffold-free approaches.
“Although being able to reproduce fundamental features of living tissues, these architectures, that often grow in an uncontrolled way, can suffer of early necrosis and batch-to-batch variability,” concluded the researchers. “To overcome these limitations, the combination of standardized additive manufactured niches and preformed multi-cellular constructs would probably be the next challenge for obtaining true biomimetic models.”
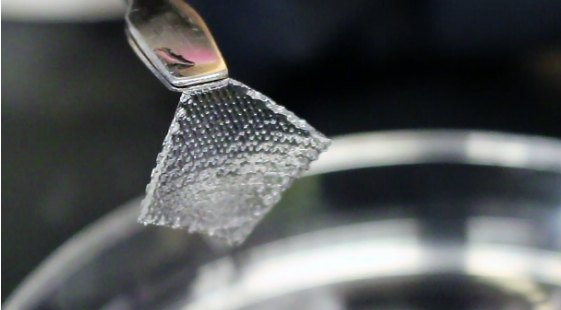
Example of a hydrogel scaffold – from ‘Bioprinting 101: Part 2 Hydrogels‘
Bioprinting only continues to show advances, from work with even more complex structures to continually new 3D printing capabilities—with the final goal being the fabrication of organs that can be successfully transplanted in a human. At that point, healthcare will change significantly as individuals receive patient-specific transplants and are able to say goodbye to both the worry of rejection as well as an interminable wait.
What do you think of this news? Let us know your thoughts! Join the discussion of this and other 3D printing topics at 3DPrintBoard.com.

What is needed to create a scaffold for tissue engineering purposes. From: ‘Bioprinting 101 – Part 11, Tissue Engineering and Regenerative Medicine‘
Subscribe to Our Email Newsletter
Stay up-to-date on all the latest news from the 3D printing industry and receive information and offers from third party vendors.
You May Also Like
Gorilla Sports GE’s First 3D Printed Titanium Cast
How do you help a gorilla with a broken arm? Sounds like the start of a bad joke a zookeeper might tell, but it’s an actual dilemma recently faced by...
Nylon 3D Printed Parts Made More Functional with Coatings & Colors
Parts 3D printed from polyamide (PA, Nylon) 12 using powder bed fusion (PBF) are a mainstay in the additive manufacturing (AM) industry. While post-finishing processes have improved the porosity of...
$25M to Back Sintavia’s Largest Expansion of Metal 3D Printing Capacity Since 2019
Sintavia, the digital manufacturing company specializing in mission-critical parts for strategic sectors, announced a $25 million investment to increase its production capacity, the largest expansion to its operations since 2019....
Velo3D Initiates Public Offering in a Bid to Strengthen Financial Foundations and Drive Future Growth
Velo3D (NYSE: VLD) has been among a number of publicly traded 3D printing firms that have attempted to weather the current macroeconomic climate. After posting a challenging financial report for 2023,...