Microneedle arrays, or MNAs, are devices made up of micron-sized needles that make it possible to transfer a signal or compound across an outer layer of tissue, like skin. Because of how tiny they are, MNAs are a fairly painless and minimally invasive device, and have applications in chemical biosensing, drug delivery, and electrical stimulation, among others. Many materials can be used to fabricate microneedles, and based on the amount of applications they can be used for, it’s important to find a more cost-effective way of making them, which is what researchers Kevin J. Krieger, Nicky Bertollo, Manita Dangol, John T. Sheridan, Madeleine M. Lowery, and Eoin D. O’Cearbhaill from University College Dublin set out to do in their paper, “Simple and customizable method for fabrication of high-aspect ratio microneedle molds using low-cost 3D printing.”
The abstract states, “We present a simple and customizable microneedle mold fabrication technique using a low-cost desktop SLA 3D printer. As opposed to conventional microneedle fabrication methods, this technique neither requires complex and expensive manufacturing facilities nor expertise in microfabrication. While most low-cost 3D-printed microneedles to date display low aspect ratios and poor tip sharpness, we show that by introducing a two-step “Print & Fill” mold fabrication method, it is possible to obtain high-aspect ratio sharp needles that are capable of penetrating tissue. Studying first the effect of varying design input parameters and print settings, it is shown that printed needles are always shorter than specified. With decreasing input height, needles also begin displaying an increasingly greater than specified needle base diameter. Both factors contribute to low aspect ratio needles when attempting to print sub-millimeter height needles. By setting input height tall enough, it is possible to print needles with high-aspect ratios and tip radii of 20–40 µm. This tip sharpness is smaller than the specified printer resolution. Consequently, high-aspect ratio sharp needle arrays are printed in basins which are backfilled and cured in a second step, leaving sub-millimeter microneedles exposed resulting microneedle arrays which can be used as male masters. Silicone female master molds are then formed from the fabricated microneedle arrays. Using the molds, both carboxymethyl cellulose loaded with rhodamine B as well as polylactic acid microneedle arrays are produced and their quality examined. A skin insertion study is performed to demonstrate the functional capabilities of arrays made from the fabricated molds.”
Important design criteria when making microneedles includes limiting needle height to the sub-millimeter range and getting the correct aspect ratio and tip radius for the needle. While MNAs can be created for direct application, the researchers chose to use them as a master for fabricating microneedle molds, which can be made from a wider variety of materials.
There are many ways to fabricate microneedles for MNA mold masters, such as UV lithography, chemical wet etching, centrifugal lithography, electrical discharge machining, and micromilling, but as most laboratories likely don’t have the necessary equipment to carry these methods out, the researchers chose to use SLA 3D printing – specifically, the Form 2 3D printer from Formlabs, using Clear Resin.
“In this study, we present a novel customizable method for fabrication of microneedle masters in the research setting, which may be used to produce female molds which aims to overcome limitations of previous microneedles produced using low-cost desktop 3D printers which often suffer from low tip radius and/or low aspect ratios,” the researchers wrote. “To improve feature resolution, we developed a two-step “Print & Fill” technique which allows for the indirect fabrication of customizable microneedle masters for mold making using SLA 3D printing. Subsequently, microneedle masters and molded microneedle arrays are dimensionally characterized and functionally assessed for reproducibility.”
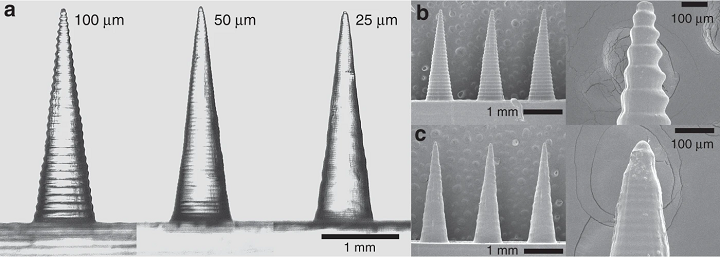
Fig. 2: Effect of layer height on print quality. a Comparison of 10, 50, and 25 -µm layer height needles; SEM images of b 100 -µm; and c 25 -µm layer height needles.
The researchers conducted a parametric study so they could find the optimal settings for fabricating the best needle geometry. In the study, they “quantified print success” by measuring needle height, angle, tip radius, and needle straightness. The needles were 3D printed at an aspect ratio of 4:1, and the team found that the smaller the layer height, the smoother the surface of the needle; acceptable needle qualities could be achieved with 25, 50, and 100 -µm, but 25 was the best.
“The first measured design parameter was the output needle height, which was seen to be lower than the set input height value. While the printed needles were shorter than specified in the virtual model, this appeared to be a predictable factor,” the researchers explained.
“A second design parameter measured was the angle θ. It is proportional to the aspect ratio for a needle with infinite tip sharpness. This value quantifies the angle between needle sides irrespective of the tip radius. The actual aspect ratio of a real needle will be lower with respect to that of a perfect needle (i.e., a needle of infinite tip sharpness) due to its inherently decreased height resulting from tip rounding. This parameter was relatively proportionate to the input value for needles of greater input height.”
The 3D printed needles’ tip radii was between 20 and 40 µm, which is sharp enough to pierce skin.
“The straightness of the needle was also quantified by extracting the needle outline from the images, converting it to a set of data points, and subsequent calculating of the coefficient of determination (R2) for the data points for each needle side. A straighter needle will have a R2 value closer to 1. Calculations found this value to lie in the range of 0.95–0.99 for all needles indicating good straightness of the print.”
The researchers used these optimized settings – 25 µm layer height, 2.5 mm input height, and 4:1 aspect ratio – as input for designing their needle array basins, which are made up of an array of 5 × 5 needles. The basins were filled with a photocurable resin in order to create four types of arrays with needles in the desired sub-millimeter range. Fabricated MNAs were used to create silicone microneedle molds, which were quality-tested by making MNAs out of two types of polymers.
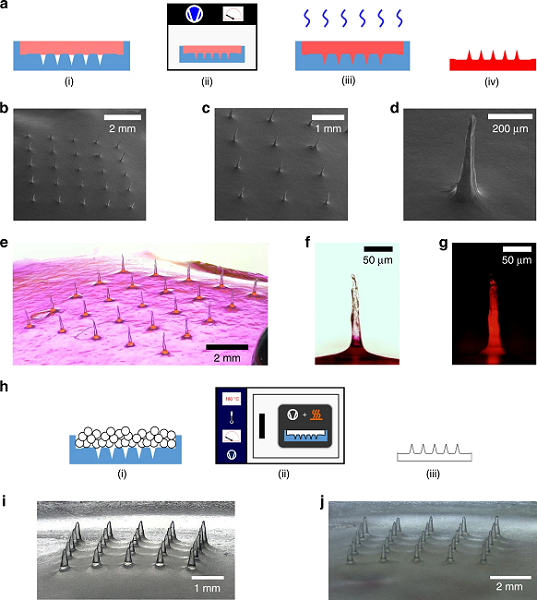
Fig. 5: Microneedle fabrication examples. a Schematic of fabrication steps of CMC MNAs loaded with Rh B: (i) fill mold with CMC-Rh B solution, (ii) place molds in vacuum chamber to remove air bubbles and fill voids, (iii) dry MNA, (iv) final CMC MNA loaded with Rh B; b–d SEM images of CMC-Rh B MNAs; e optical image of CMC-Rh B MNA; f optical image of individual Rh B-loaded CMC microneedle; g optical fluorescence image of Rh B-loaded CMC microneedle; h PLA microneedle casting using mold: (1) mold filling with PLA pellets, (2) placing of mold with pellets in vacuum oven, (3) demolding to obtain PLA MNA; i replica molded PLA MNA from master MNA; j replica molded PLA MNA from master MNA.
The researchers used the molds to create PLA microneedles, and also conducted a successful porcine skin insertion study with the PLA MNAs.
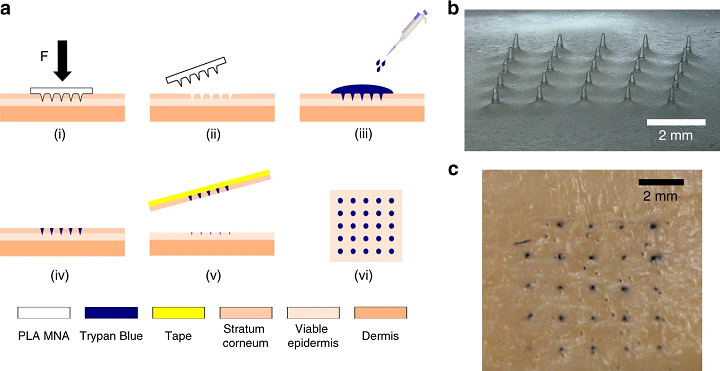
Fig. 6: Skin insertion study. a Schematic representation of skin insertion test: (i) MNA insertion into skin applying a force of 30 N for 60 s, (ii) MNA removal, (iii) trypan blue staining left for 120 s, (iv) washing off trypan blue with 0.9% saline solution, (v) tape-stripping of the stratum corneum from the skin, (vi) inspection of sample for trypan blue stains; b replica molded PLA MNA (mold master: 1.5 mm needle-to-needle distance and 600 mg fill weight); c porcine skin sample after MNA insertion and staining.
The team’s two-part, inexpensive, and customizable Print and Fill method can definitely be used by other microneedle researchers in order to complete in-house fabrication of master molds and parametric optimization of MNAs.
Discuss this and other 3D printing topics at 3DPrintBoard.com or share your thoughts below.
Subscribe to Our Email Newsletter
Stay up-to-date on all the latest news from the 3D printing industry and receive information and offers from third party vendors.
Print Services
Upload your 3D Models and get them printed quickly and efficiently.
You May Also Like
Reinventing Reindustrialization: Why NAVWAR Project Manager Spencer Koroly Invented a Made-in-America 3D Printer
It has become virtually impossible to regularly follow additive manufacturing (AM) industry news and not stumble across the term “defense industrial base” (DIB), a concept encompassing all the many diverse...
Inside The Barnes Global Advisors’ Vision for a Stronger AM Ecosystem
As additive manufacturing (AM) continues to revolutionize the industrial landscape, Pittsburgh-based consultancy The Barnes Global Advisors (TBGA) is helping shape what that future looks like. As the largest independent AM...
Ruggedized: How USMC Innovation Officer Matt Pine Navigates 3D Printing in the Military
Disclaimer: Matt Pine’s views are not the views of the Department of Defense nor the U.S. Marine Corps Throughout this decade thus far, the military’s adoption of additive manufacturing (AM)...
U.S. Congress Calls Out 3D Printing in Proposal for Commercial Reserve Manufacturing Network
Last week, the U.S. House of Representatives’ Appropriations Committee moved the FY 2026 defense bill forward to the House floor. Included in the legislation is a $131 million proposal for...